There must be a theory hidden from our eyes which will allow us to easily calculate everything in the universe. That’s the theory we are looking for, says professor Grzegorz Wrochna in conversation with Robert Siewiorek
Robert Siewiorek: Richard Feynman, a Nobel Prize winner in physics, once said: “You don’t really understand something, unless you can explain it to your grandmother.”Let’s say I’m your grandma asking you what you actually did in CERN.
Grzegorz Wrochna*:This quote is a motto for all my research work. I’ve always tried to write scientific articles and deliver lectures to make them easy to understand not only for grandmas but even for kids. Feynman may have exaggerated, but only a bit. I once tried to explain what we did in CERN in a radio program. Several days later I met a physics teacher who brought her whole class to listen to my lecture.“You know,” she said, “I got a call from my mom who had listened to your program and said: ‘I have finally understood why you take your pupils to that CERN thing.’”
OK, let me ask you a serious question now: If we treated the general theory of relativity and quantum mechanics literally and if we imagined what the universe should look like, then, as Harry Cliff, a physicist working with the Large Hadron Collider, put it, the universe should be boring, menacing and lifeless. But it is exactly the opposite: it’s fascinating, full of stars and squirrels. Why is there something rather than nothing?
Both scientists and grandmas have equal chances to come up with the right answer. It’s a philosophical question going way beyond physics and science and none of us can even get close to solve that conundrum. The theories you’ve just mentioned are mere models describing that part of the world we have managed to explore and understand. Although not fully. That’s why we are still looking for new theories that would describe increasingly more complex phenomena and that would help us understand the evolution and the laws of the universe. But even if we finally get there, there will be no answer to your question.
Let’s get back to the starting point in the history of the universe: there is completely nothing and suddenly you can hear a big “bang!”.One ten-billionth of a second after the explosion something is created. It’s a substance, a driving force giving birth to the matter. What happened exactly?
Let me start with the basics. All matter consists of atoms, atoms are composed of nuclei made from protons and neutrons, with electrons orbiting around them. Everything that exists – inanimate and animate nature, including ourselves – is made from those particles, i.e. elementary particles (there are also light-carrying photons and other electromagnetic waves).These particles and laws they follow make the universe complex and beautiful. We physicists are trying to understand it. Over one hundred years ago the question arose as to whether protons and electrons consist of even smaller particles. And it has turned out that they do.
How do you know that?
In order to see what’s inside a walnut you need to use a nutcracker to crack the husk. And if you don’t have a nutcracker, you can always hit walnuts against one another. And that’s exactly what we do in the CERN Large Hadron Collider. We accelerate protons to an enormous speed and make them hit one another hoping they will split into smaller particles.
Any success?
It has been proved that protons and neutrons consist of smaller particles called quarks, which unfortunately cannot be split permanently. Quarks cannot exist independently. They immediately form other particles which are sometimes exotic and made from two or more quarks. Recently CERN has discovered one of them.
Tetraquark?
Yes. It’s a meson comprising four quarks. Before the Large Hadron Collider was created, a whole range of such particles had been discovered that had been hard to analyze back in the day. But eventually the physicists have managed to develop a theory called the Standard Model of elementary particles, which accurately explains where such particles come from.
When the physicists wrote down the equations relating to quantum physics and the general theory of relativity to describe the universe, they got a beautiful model with one major flaw: the particles had no mass. And that kind of world wouldn’t exist
The history of physics can be described as consecutive attempts to unify different interactions. Let’s take a look at gravity, for example. Newton not only discovered that gravity makes objects fall on Earth but also that it makes planets revolve around the Sun. Two utterly different phenomena, i.e. the falling of the legendary Newtonian apple and the motion of the planets, were the result of the same interaction. The same goes for the electric and magnetic fields; people thought they were two different fields but the progress in physics has made us realize that they are two ways manifesting the same interaction.
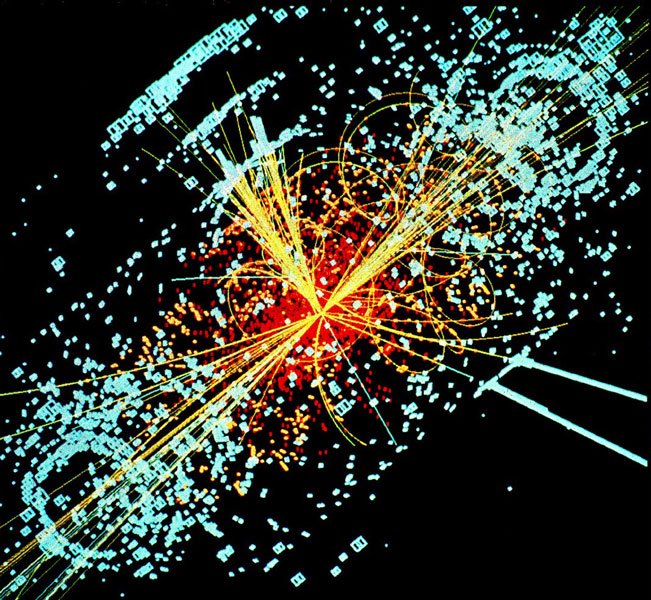
As a result, today we know four basic interactions: electromagnetic, gravitational, plus two more nuclear interactions: strong and weak. Electromagnetic and weak interactions are two manifestations of the so-called electroweak interaction, but this unity can be seen only in the case of very big energies, like the ones generated in CERN during the previous generation experiments. The standard model cohesively describes strong interactions (only the gravitation is currently set aside).
If that model is so great, what is the problem?
When the physicists wrote down the equations relating to quantum physics and the general theory of relativity to describe the universe, they got a beautiful model with one major flaw: the particles had no mass. And that kind of world wouldn’t exist.
So what did they do?
They used an idea of British scientist Peter Higgs; they supplemented the equations with a part that adds mass to particles.
As simple as that?
Yes, call it a brutal operation if you like. And suddenly everything started to make sense.
Can you even do that?
Well, if those equations describe the real world, the added element must have an equivalent in the real world. This equivalent is a new particle which, named after professor Higgs, is called the Higgs particle (Higgs boson), also sometimes referred to as the “God particle”.
What is godly about it?
Nothing. The term was coined to make the book in which the phenomenon is described sell better. That’s all. But one has to admit that from the perspective of our whole knowledge about the universe Higgs boson is key, because it adds mass to all particles. Without it, the world would be really boring, empty and devoid of squirrels.
And then year 2012 came…
Yes. After many years, the Large Hadron Collider in CERN finally made it possible to generate the Higgs particle.
Does that mean that the Large Hadron Collider was created to replicate the conditions existing at the beginning of the universe, at the moment of the Big Bang? Is it used for making many small Big Bangs?
I guess you can say that.
That would mean that scientists are imitating the act of creation so they are playing God. Am I right?
No. Considering the Big Bang equivalent to the moment of creation of the world is a stretch because we don’t know the whole history of the universe starting at point zero. Our theories can describe the evolution of the universe as from that one ten-billionth of a second after the Big Bang. So it’s easy to neglect everything that happened before.
“Everything”? Are you suggesting that so many things could have happened in that tiny fraction of a second?
Yes. In the context of the history of the universe, the closer to the Big Bang, the shorter the eras in which great changes took place. Although the formation of stars and planets took billions of years, the first three minutes, when the first atomic nuclei were created, were vital for the universe.
Considering the Big Bang equivalent to the moment of creation of the world is a stretch
We don’t know whether between point zero and that one ten-billionth of a second there were ten or more eras in which unknown and extraordinary things happened. That’s what we analyze in the Large Hadron Collider. And if it doesn’t help us to discover anything new, we will have to build bigger colliders to reach even bigger energies.
It has already been planned. One is to be constructed in Europe, the other one in China. Both of them are going to be bigger than the one close to Geneva, each of them with the circumference of one hundred kilometers. Do they have to be that big?
Yes if we want to reduce the time of one ten-billionth of a second to one twenty- or one fifty-billionth of a second and to discover new phenomena.
What for?
The standard model may describe the phenomena we know with the highest possible accuracy, but it has two flaws. Firstly, it doesn’t describe the gravity. Einstein devoted most of his life to that; many eminent scientists have still been working on it, but so far with no success. Besides, as far as physics is concerned, the simplest and the most beautiful theories prove to be true.
It is said that the Higgs boson is not beautiful.
That’s the point: the standard model is ugly.
What do you mean “ugly”?
As far as the fundamental theory goes, we expected all numbers, data, masses of various particles and laws they follow to result, in terms of numbers, from that theory. We were aware that we might add to it one or two fundamental constants to give us the image of the real world but not more than that. But it turns out that the standard model has to be supplemented “manually” with about 20 parameters. For example, we are unable to estimate the mass of particles. You can say that the Higgs mechanism is attached to the model with a piece of adhesive tape as it does not result from the theory.
Does it work?
It does but the scientists believe that it’s a stopgap as there must be a theory hidden from our eyes which will allow us to easily calculate everything in the universe. That’s the theory we’re looking for.
How? By achieving even higher levels of energy?
Yes. Hoping for new particles to be generated and new interaction features to appear.
Is there any other way to get to that theory?
Yes, it’s searching for very rare phenomena in which the results of the standard model will be breached, broken. But that will take us some time too. Today, at virtually every conference, physicists announce they have come up with a result that slightly diverges from their expectations. Except that, at the next conference, it is proven that it doesn’t.
How did you get to CERN?
Being a student of the second year at the Faculty of Physics, University of Warsaw, I attended a seminar held by professor Janusz Zakrzewski called “e+e-“. It was about colliding electrons with antielectrons. Back then, experiments of that sort were conducted in DESY in Hamburg, which was one of the biggest research centers in Europe and which, at that time, owned the second largest particle accelerator. After the second year of my studies I went to Hamburg to participate in preparations for some experiments. Working there was fascinating so I decided to follow that path.
After my third year I went to CERN to serve a two-month student internship (I was in a group with Fabiola Gianotti, who was a PhD student then and who now is the Director-General at CERN). When I defended my PhD thesis supervised by professor Andrzej Wróblewski, first talks about how to build the Large Hadron Collider and what experiments could be done with it started. Three of my older friends went to the conference where those issues were discussed. They all agreed that Polish teams would contribute the most if they joined the experiment called CMS done with the use of a huge magnet measuring six meters in diameter. We were all welcome but there was one condition: one of us had to work stationary in CERN. And that was me. I defended my PhD thesis on Friday. On Saturday I threw a farewell party and on Sunday I was already in CERN. I spent there seven years. When I came back to Poland, I still was a member of that team and I worked with them. In total, I was involved in CERN projects for 18 years.
What did you do?
My job consisted mainly in designing the CMS experiment. It was about computer simulations in which the particles created after collision between protons were tracked during their travel through particular elements of the detector. Particle paths and signals of electronic devices recording the movement of particles were calculated. The results were used to determine the size of detectors, materials they should be built from and the degree of accuracy of measurements. All this was done to register the particles that would confirm, for example, the creation of the Higgs boson or any other exotic particle in the most effective manner possible.
After the Higgs boson was discovered on 4 July 2012, you said that it was the biggest contemporary achievement of the humanity, surpassing the Moon missions. Why is it so important?
Without the Higgs particle the world would not exist. Interestingly, the existence of this particle was suspected long before the experiment. Before it was finally materialized, several generations of colliders had been constructed with the aim of discovering it.
Gian Giudice, your colleague from CERN, said that we are just travelers who put up their tent at the edge of a cliff. The problem with the Higgs particle is that a slight disturbance in its mass is enough to make everything fall apart.
Our world is unique. The world, the planets and the squirrels can only exist if many physical parameters have a certain precise value given to a specific number of decimal places. People who are fascinated by it ask themselves what would happen if the value of any of those decimal places was changed. In consequence, there is a question if those numbers are chosen by God to make it possible for humans to appear. This is the so-called anthropic principle. Or maybe it’s the other way round? Maybe there is a whole raft of worlds with each of them having different numbers and we live in one into which, for one reason or another, we have been thrown?
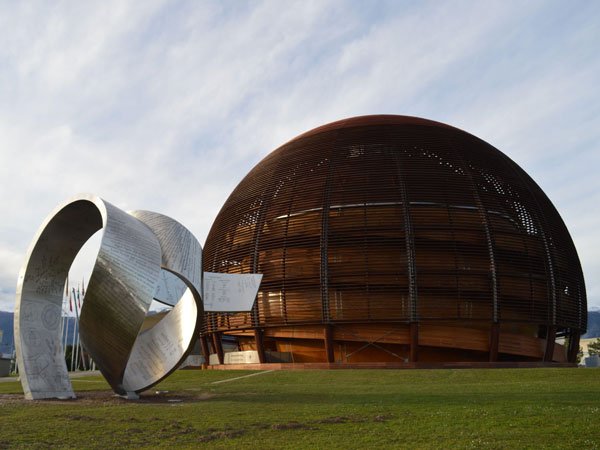
Would this imply that it was God who decided about the mass of the Higgs particle?
Yes, but it’s hard to believe that God tinkered with various physical constants helter skelter. It is more likely that he designed all laws of physics consciously to make everything work like a charm.
If you consider a possibility that it might have been the other way round, it also means that you do not reject the theory of multiverse – a structure in which our universe is a bubble in the ocean of other bubbles where there are seemingly same laws which are not applied in the same way. Am I correct?
Theoretically, that possibility cannot be excluded, but it doesn’t solve the problem of the anthropic principle and the fact that all numbers we have mentioned are so precise. One of the pre-conditions of the multiverse with many dissimilar universes of different characteristics is to properly set all the parameters. Random parameters could lead to nothing rather than something.
The multiverse was once compared to dunes. Since there are millions of dunes, each of them should be different but due to the laws of physics their slope angle all around the world is always 30 to 35 degrees. Would the laws of physics work in the multiverse in a similar way?
Yes. If we accept this hypothesis, we should no longer ask about the numbers or values of physical constants but about the shape of the laws of physics. But that is no solution to the problem; it’s making it more elegant.
How do you use artificial intelligence in CERN?
Mainly to interpret data gathered during experiments. We are not able to see the Higgs particle because its life is too short to be recorded by any detector.
So how do you know it exists?
Because we can calculate into what other particles it splits. It can split in two photons or four particles called muons, which we are able to record.
When two protons collide a lot, sometimes even thousands, of particles are created and we record their paths, analyze the curve of their trajectories in the magnetic field, which allows us to determine their energy. Then, we store them in detectors called calorimeters where their energy is converted to a bunch of electrons and photons which we can measure and with respect to which we can determine the energy of particles. Using all those measurements – it’s several million numbers per event– we are trying to infer what happened.
As far as physics is concerned, the simplest and the most beautiful theories prove to be true
But all this wouldn’t be so complicated if it wasn’t for the fact that phenomena like creation of the Higgs boson are extremely rare. To find the Higgs boson, you need to collide protons every twenty-five-billionth of a second (in fact we collide whole “packages” of protons). Every second we provoke a huge number of collisions and we do that almost continuously throughout the whole year. What’s interesting is that there are no more than several hundreds of cases where the Higgs particle can be observed (depending on what it is split into). It’s like looking for a needle in all haystacks around the world.
And AI is used to help you find it, right?
Mostly. First trials were conducted with neural networks that were trained in simulations. Knowing, in theory, what particles the Higgs boson should be split into, we can model the answer of a detector to the appearance of such particles. Data collected from millions of similar cases are used to train neural networks to analyze subsequently observed cases.
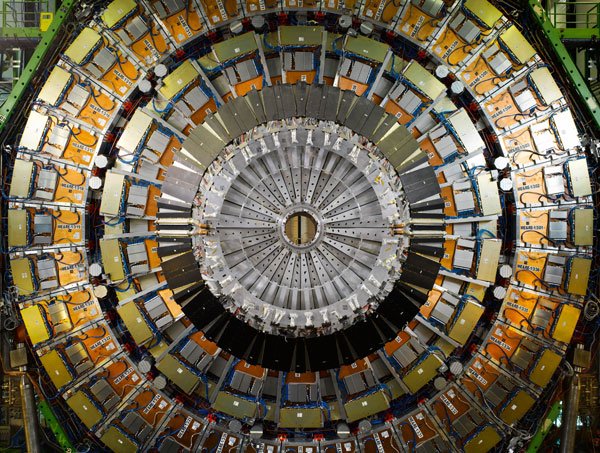
Other than that, AI proves useful in diagnostics and fixing CERN detectors which are gigantic devices several stories high. If anything breaks down, it is hard to disassemble the whole thing to look for a failure. This is why we use augmented reality: 3D goggles display a digital clone of a detector along with its virtual plan where you can see what is wrong. The technology was first developed for the purpose of CERN research but it was later applied for many other things, for example in therapies for autistic children.
Do you rely only on artificial intelligence?
No, we combine it with standard methods of statistical analysis. Verification is a must. After all, there is only one Large Hadron Collider in the world. Other than that, we use it to conduct two big experiments which verify one another. Additionally, in each of them, we are trying to prove that the Higgs boson exists and to analyze its properties.
Do you think that artificial intelligence has a future in physics of particles?
Of course. AI will prevail wherever there is a huge amount of data.
It is said that every hour the Large Collider generates more data than Facebook in a year.
That’s true. We’re talking about an enormous amount of data. And the more data we have, the less effective standard analytical methods get. The progress of physics of particulars, construction of increasingly bigger accelerators, use of increasingly greater energies and newer experimental methods result in an increasing amount of data from experiments. If those huge, one hundred kilometer colliders in Europe and China are built, it will be even harder to interpret data generated during experiments done in those devices.
Why?
For example because one collision will involve not hundreds but tens of thousands of different particles. And artificial intelligence simply loves big data.
Did you also use artificial intelligence in “Pi of the Sky”?
I worked on that project together with professor Lech Mankiewicz from the Center of Theoretical Physics of the Polish Academy of Sciences. We used a highly specialized camera to take photos to discover the so-called gamma-ray flashes. In the universe there are many cataclysms happening on a large scale, including supernova explosions, star mergers etc. Those phenomena were observed by satellites recording gamma radiation, which doesn’t reach the Earth. The problem is that radiation detectors cannot accurately determine the direction where from it comes. For that reason it was difficult to say what object emitted it. Therefore, the same part of the sky has to be also observed with a standard telescope to capture light radiation as well. Only then can you determine where the object that emitted gamma radiation is. But all this has to be done extremely fast and large telescopes require even up to tens of minutes to orient towards relevant regions of space.
Do you have to predict the location where from radiation may come?
Yes, but that it is impossible. The idea of the project was to focus a telescope towards a place where an explosion is to happen before the flash actually occurs. We observed a large part of the sky to correlate the flash we were going to observe with a signal from the satellite. There is a whole lot of such flashes in the sky: there are variable stars, meteors penetrating the atmosphere of Earth, elementary particles that come from space, and “glints” caused by light reflected from the surface of satellites.
Our world is unique. The world, the planets and the squirrels can only exist if many physical parameters have a certain precise value given to a specific number of decimal places
To distinguish one from another AI is very convenient. But when we worked on “Pi of the Sky”, AI’s methods were not as advanced as they are today. That’s why the results we got were interesting but not as good as the ones obtained with the use of standard methods.
What do Poles do in CERN now?
They participate in experiments done with the Collider, collect and analyze data. As the data gathered in previous years are still being analyzed, they are involved in that project too. And, thirdly, the accelerator and the detectors are constantly being improved (it is possible to force particles to collide with each other more frequently than until recently). We contribute to every part of CERN activity.
What are the Collider’s benefits for an average Joe?
Main research in CERN is done in parallel with development of technologies for accelerators and detectors used in medicine. For example, proton radiotherapy to fight cancer is performed with the use of cyclotrons, i.e. accelerators in which particles are accelerated along a spiral path and oriented towards a tumor only after having reached a desirable speed. CERN is also working on a linear accelerator that should be at least two or three times cheaper, making the therapy more accessible.
Anything else?
Yes. The crystals that were developed for the Large Collider to record photons into which the Higgs particle splits are used in positron emission tomography to scan brains, hearts and tumors. Many discoveries made in CERN have been implemented in medicine and economy.
Internet, for example.
Yes. The internet, or, to be more precise, the http protocol and the html language, was created in CERN to serve the needs of the Large Hadron Collider and LHC research.
What is the most important question you are asking yourself as a scientist?
I think that the most interesting thing is how this beautiful theory describing all phenomena we know today looks like.
The theory of everything?
That’s the common name for it although, to be more precise, it is about the theory of the physical world. Today we live in a state of schizophrenia. We have two theories: quantum mechanics, which we have managed to reconcile with the Einstein’s special theory of relativity but not with the theory of gravity, or the Einstein’s general theory of relativity. Both theories are excellent and indispensable. For example, we need the latter for GPS to work. On the other hand, the world of computers, information technology, semi-conductors – all this is based on quantum mechanics. Both theories have proved solid but they work in different areas: the theory of gravity on a large scale and mechanics on a small scale – and they are contradictory. Physicists have been trying to reconcile them for one hundred years but so far to no avail.
Why do you love classical music so much? Is it your way to escape a complicated world of contradictions to the land of simplicity? Does it help you find peace and harmony?
I am fascinated with the Middle Ages, a fantastic epoch, in which the first universities were founded and contemporary science began to thrive. I admire the time when people did not have to confront the dilemma of whether science contradicts faith and when they could reconcile both. This music has the spirit that combines those two worlds instead of dividing them. It is relaxing and soothing and allows me to distance myself from chaos and haste.
But that is not the only music I like. I like any kind of good music.
*Grzegorz Wrochna, undersecretary of state in the Ministry of Science and Higher Education, professor of physical sciences. In 1991–1998 he was involved in designing and constructing the CMS experiment with the CERN Large Hadron Collider. In 1999 he became the director of the Institute of Nuclear Problems; he initiated the establishment and was later appointed the director of the National Center for Nuclear Research. He has represented Poland in EURATOM program committees (since 2007), in the Supervisory Board of European XFEL in Hamburg (since 2009 r.), in the Supervisory Board of the EU Joint Research Centre (since 2019). Between 2017 and 2018 he was the chairman of the Committee for Scientific Policy and between 2015 and 2016 he was the head of the European Atomic Energy Society. In 2013–2019 he was a member of the management board of the European Sustainable Nuclear Energy Technology Platform (SNETP) and since 2015 he has been the chairman of the Nuclear Cogeneration Industrial Initiative (NC2I). In 2015–2018 he was also a member of the “Nuclear Innovations 2050” advisory committee of the OECD Nuclear Energy Agency. He likes hiking, swimming, science-fiction literature and good music.
Przeczytaj polską wersję tego tekstu TUTAJ